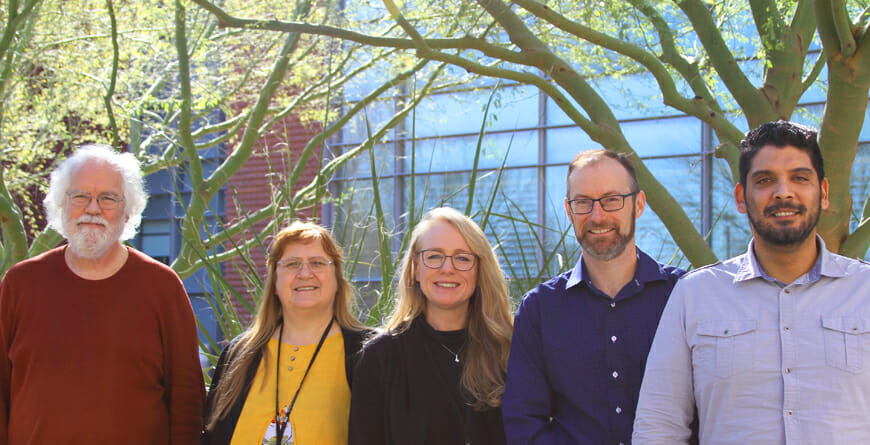
The quest for sustainable energy has become a central challenge for society. In order to meet ever-expanding energy demands without further damaging the global climate, researchers are tapping into natural processes that have provided plants and many animal forms with their energy source for billions of years. Their secret is the conversion of radiant light energy into chemical energy in the process of photosynthesis.
In new research appearing in the current issue of the Journal of the American Chemical Society, lead author Christine Lewis and her ASU colleagues describe a patented hybrid device—part living organism, part bio battery, capable of producing stored energy by increasing energy flow under light conditions where natural photosynthesis is normally inhibited.
The advancement of such technologies offers a green pathway to the production of a broad range of useful products, including transportation fuels, agrochemicals, therapeutics, cosmetics, plastics and specialty chemicals as well as human and animal supplements.
The new study shows that modified photosynthetic microbes—in this case, cyanobacteria— can be fed electrons from an external source and use these to power chemical reactions that could eventually be harnessed for human applications. Researchers call this approach microbial electro photosynthesis or MEPS.
“This project involves unlocking the mysteries involved with energy transfer. Specifically, we work on bridging artificial energy with natural photosynthesis by tapping into the latter half of the photosynthetic electron transport chain,” Lewis says. “The research objectives are to have the ability to turn photosynthesis on at will, eventually to make it more efficient, and produce stable energy products.”
Lewis is a researcher in the Biodesign Center for Applied Structural Discovery (CASD), Swette Center for Environmental Biotechnology (EB), and ASU’s School of Molecular Sciences (SMS).
She is joined by ASU colleagues Petra Fromme, director of the Center for Applied Structural Discovery; Bruce Rittmann, director of Swette Center for Environmental Biotechnology and professor from ASU’s School of Sustainable Engineering and the Built Environment; Wim Vermaas from ASU’s School of Life Sciences and Julie Ann Wrigley Global Institute of Sustainability (GIS); Cesar Torres from EB and ASU’s School for Engineering of Matter, Transport and Energy; Justin Flory, associate director for Engineering Center for Negative Carbon Emissions and Thomas and Anna Moore, from GIS, SMS and CASD.
Photosynthesis 2.0
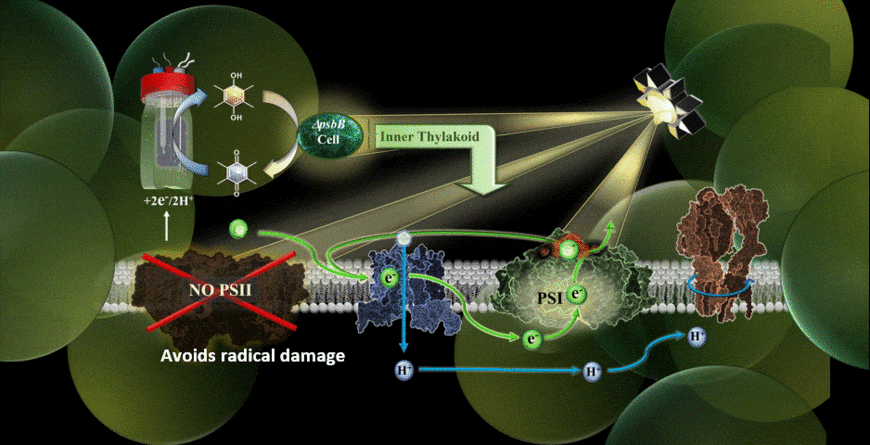
The basic recipe for natural photosynthesis involves just a few key ingredients: water, sunlight, and CO2. Photosynthetic cells act as tiny factories for the production of glucose, which is then converted into ATP, the cell’s primary energy currency. In the process, oxygen is produced as a respiratory byproduct but can prove harmful to the photosynthetic process when damaging oxygen radical species are produced with high-intensity light.
Although photosynthesis is ideally suited to supplying the energy needs of plants and other photosynthetic organisms, the rate with which light is converted into useful chemical energy is far too low to be suitable to supply today’s human energy needs. Researchers have long sought out ways to tap into natural photosynthesis while also improving it to find carbon neutral energy solutions.
Partnering with nature
There are several important limiting factors in terms of energy conversion efficiency in natural photosynthesis. First, photosynthetic organisms use only a small portion of the spectrum of light emitted by the sun, namely red visible light. Second, the rate of carbon fixation is too slow for practical applications. Increasing it requires a boost in the rate of electrons moving through the transport chain.
Finally, photosynthetic organisms can only deal with a limited quantity of sun-excited electrons at one time. If the electron transport chain is fed too many at once, the process can shut down due to light damage, disabling or killing the cell. This limitation on energy efficiency is primarily due to a key component in the cell’s electron transport machinery, a protein complex known as photosystem II (PS II).
In the new study, the MEPS system is described using a genetically modified cyanobacterium hitched to an external cathode. The cyanobacteria used were reengineered in the laboratory of co-author Wim Vermaas to carry out photosynthetic cycling of electrons without a photosystem II component.
With the help of chemical mediators, electrons are shuttled from the device’s cathode into the electron transport chain of the cyanobacterium. Because the light-vulnerable photosystem II has been eliminated, the photosynthetic process takes place via an alternate pathway, namely through photosystem I.
The results verified that photosynthesis can indeed be carried out using an external supply of electrons feeding the electron transport chain, and it could be performed in the presence of extremely high-intensity light.
“One of my priorities as part of the team was finding the right electrochemical mediator to move electrons into the cell,” Torres said. “I think that one of the highlights was realizing we have alleviated some of the bigger limitations of Synechocystis (cyanobacteria) removing photosystem II for the system and giving them electrons from an electrode.”
Sustainable futures
The MEPS system could potentially use currently available solar cells to provide the external electrons needed to power photosynthetic reactions. Photovoltaics could supply electrons from wavelengths from zero all the way up to thousands of nanometers, providing a much broader spectrum for light harvesting than usually available to natural photosynthesis.
The project, six years in the making, represents a melting pot of scientific disciplines, including microbiology, engineering, biochemistry, electrochemistry, photochemistry, and physics. It has been the focus of considerable excitement following Lewis’ presentations at a variety of conferences and her research has garnered a number of important awards, including the 2021 North American International Society of Electrochemical Microbes Conference Best Oral Presentation Award, the 2021 Eastern Regional Photosynthetic Conference Best Poster Award, the 2019 Nature Conference Energy Award, the 2019 Gordon Research Conference award and the 2018 Madame Curie Award at Biodesign’s Fusion retreat.
“By the year 2050, with global expansion moving at the pace that it is, our energy needs will surpass our supply. However, we can act now to learn how to provide efficient and cleaner energy,” Lewis says. “It is my goal to contribute to the next “breakthrough” that will help to make this big, blue marble a better place."