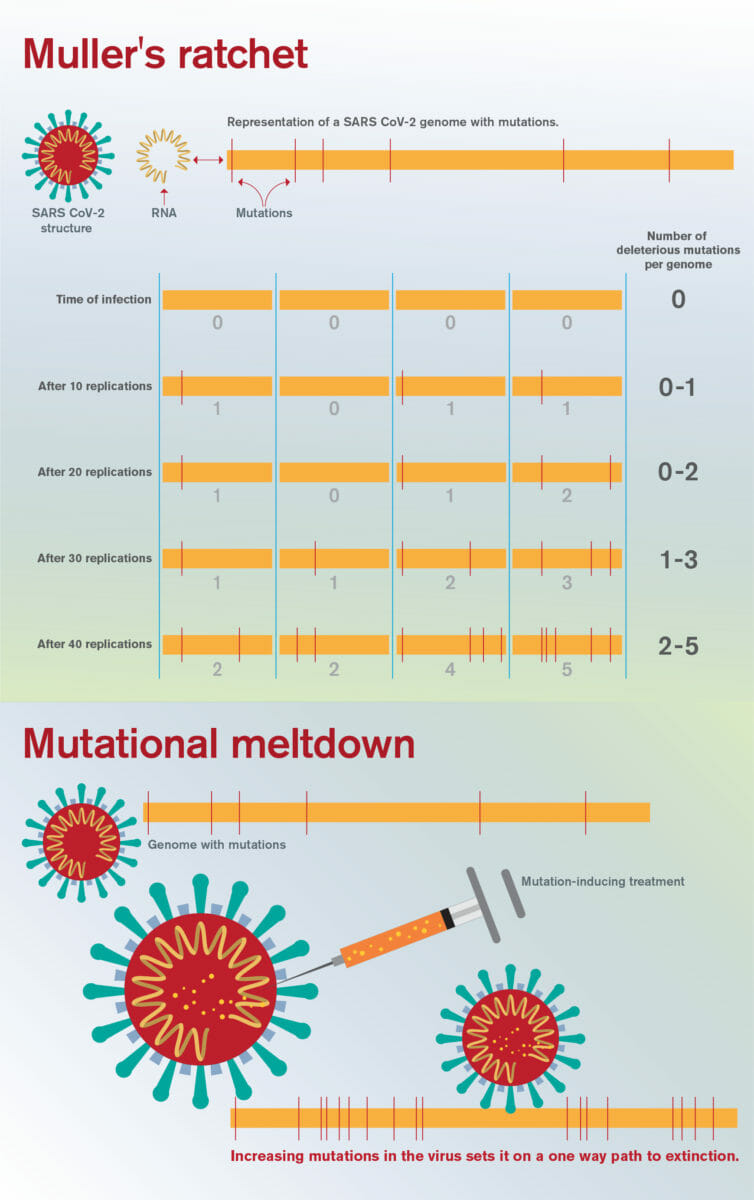
From New York to Luxembourg, Namibia, Iceland and Bhutan, the novel coronavirus SARS CoV-2 has turned the modern world into a crisis zone. An unprecedented global effort is underway to understand the elusive pathogen and find effective therapies.
An intriguing approach to treating Covid-19, the disease caused by the emergent virus, has recently been suggested by Arizona State University faculty members Jeff Jensen (Center for Evolution and Medicine) and Michael Lynch (Biodesign Center for Mechanisms of Evolution).
The strategy they describe for vanquishing SARS CoV-2 is rooted in evolutionary theory. The basic idea is simple: If a particular drug could sufficiently boost the virus’ rate of mutation, it may be possible to saddle the pathogen with so many harmful genetic errors that the viral population within a patient, collapses.
The phenomenon, known as mutational meltdown, was first described by Lynch three decades ago in the context of preventing extinction in small or endangered populations.
In the new research, the goal instead is to encourage extinction in large viral populations, through a ruinous accumulation of viral mutations. (The concept is similar to a later-described phenomenon known as lethal mutagenesis.)
“This is a great example of well-established principles in evolutionary theory being repurposed for solving significant practical problems,” Lynch says.
In addition to providing a hopeful candidate for the treatment of SARS CoV-2, mutational meltdown could offer a new blueprint for treating a broad range of infectious diseases. The method is particularly attractive as it holds the potential to deliver a knockout blow to infectious agents, causing such widespread damage to their genomes that they are unable to develop antiviral or antibiotic drug resistance.
Lynch and Jensen recently outlined the strategy in the journal Heredity.
In earlier proof-of-concept research, Jensen, his postdoctoral associate Claudia Bank (now a faculty member at the University of Berne, Switzerland) and their colleagues at the University of Massachusetts Medical School demonstrated that the drug favipiravir, if given at sufficiently high dosage, could effectively induce mutational meltdown in laboratory populations of the influenza A virus.
New killer on the block
As of May 14, 2020, confirmed cases of Covid-19 number over 4.5 million globally. Over 300,000 have died and the numbers almost certainly represent a significant undercount.
The success of SARS CoV-2 in invading human cells and rapidly disseminating through the global population is due to the virus likely having undergone specific mutations, allowing it to make a leap from some reservoir species to humans. (Such species-straddling pathogens are called zoonotic.)
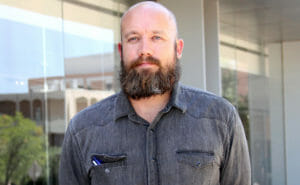
Genetic mutations are nature’s ultimate double-edged sword. They provide the raw material for evolution to act upon, and account for the astonishing diversity observed across species, including humans. Through natural selection, mutations conferring an adaptive advantage in nature’s great game can become fixed in a population and transmitted to offspring through generations.
Most genetic mutations, however, are deleterious and these account for a dizzying range of diseases, from mild to lethal. Over successive generations, natural selection can act on these errors, increasing the probability of ultimate fixation for mutations that are advantageous, and decreasing the probability for those that are deleterious owing to the reduced biological fitness of organisms carrying these harmful mutations. (Fitness is a measure of an organism’s ability to grow to adulthood and successfully contribute progeny to the next generation.)
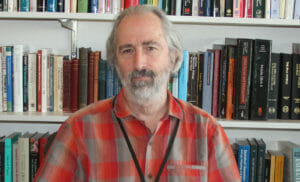
While harmful mutations can wreak havoc in individuals who acquire them, over time, populations tend to shed the majority of detrimental mutations. In organisms that reproduce sexually, this purging process is helped along by the recombination of genetic elements provided to offspring from two genetically distinct parents.
Ratchet of doom
Although evolution acts to remove deleterious mutations from the population through purifying selection, there are limits to this cleansing process. Should the overall population number fall below a certain threshold, purifying selection can’t keep pace with the accumulation of bad mutations.
“This is because the efficiency of selection is reduced in small populations, owing to the increased noise in the transmission process across generations,” Lynch says. In this case, biological fitness in the remaining population will be continually degraded, placing the organism on a one-way path to extinction.
For example, a population initially bearing no harmful mutations will eventually degrade to a population with all members bearing at least 1 bad mutation, later, 2, then 3 and so on. Such populations grow successively sicklier over time, further reducing population size, in a snowball effect.
This irreversible mechanism, long recognized by evolutionary biologists, is known as Muller’s ratchet, for the ratchet-like, one-way accumulation of harmful mutations in a population. This process is traditionally viewed as being the result of genetic drift, a random evolutionary process, but under high mutation rate regimes these 'clicks of the ratchet' may be driven by mutation itself.
Hacking the viral genome
Typically, single-stranded RNA viruses have a 100-1000-fold higher mutation rate per replication than humans do per human generation. Coronaviruses like SARS CoV-2, however, are unique among RNA viruses as they possess a proofreading domain used to reduce mutational accumulation.
The strategy outlined seeks to target this proofreading apparatus through drug therapy, damaging its functionality. If the virus could be induced to pick up deleterious mutations at an accelerated pace, Muller’s ratchet may begin clicking, eventually reducing population fitness to the point of no return: mutational meltdown.
"Practically, a key question here is how much the mutation rate must be turned up to achieve this effect," Jensen noted, "as one certainly doesn't want to run the risk of an insufficient rate increase that may generate adaptive mutations without generating a sufficient deleterious mutational load to ensure extinction."
If a viral invader like SARS CoV-2 were treated with a mutation-boosting drug at sufficient dosage, the result would be to shower the entire viral genome with mutations, which could make it very hard for the virus to evolve resistance.
The drug favipiravir is one such mutation-enhancing candidate, which has already shown promise in this regard in a number of RNA viruses, including Ebola, yellow fever, chikungunya, enterovirus and norovirus.
In the previous work on influenza A virus, Jensen and colleagues accurately predicted the subtle dynamics of mutational meltdown due to favipiravir, which involved a linear rate of mutational accrual leading to a fatal transition point, beyond which harmful mutations rapidly accumulated prior to the collapse of the viral population.
Recently, another drug, remdesivir, has shown promise against a clinical isolate of SARS-CoV-2. Like favipiravir, the treatment appears to confuse the virus’ genetic proofreading system. As Lynch notes, “anything we could do to muck up the proofreader would increase the mutation rate around 20-fold.”
Such therapies could be used in conjunction with other drugs targeting different aspects of viral assembly or replication, further increasing the likelihood of achieving effective meltdown. Much more work is needed however - both experimental and theoretical - to determine if the strategy can be safely and effectively applied to treat Covid-19, or indeed be used as a general anti-viral strategy in future unknown pandemics.